Utilizing Soil Moisture Sensors for Efficient Irrigation Management
DOWNLOADApril 22, 2025 - Angie Gradiz and Younsuk Dong , Michigan State University Extension
Effective irrigation management is essential for sustainable agriculture and improving yields, water efficiency, and returns on investment. Advanced technologies such as soil moisture sensors help inform irrigation decisions by measuring soil water content so that the right amount of water is applied at the right time. Choosing the right sensor depends on factors like cost, reliability, durability, and ease of use. This fact sheet outlines key types of soil moisture sensors, costs, and best-use recommendations.
Soil water content basics
Soil moisture sensors provide indirect measurements of soil water content, typically through volumetric water content (θv) or matric potential (ψm). Volumetric water content represents the ratio of water volume to the total volume of soil, while matric potential indicates the pressure a plant must exert to extract water from the soil. Key soil moisture terms include saturation, where the soil pores are saturated with water, field capacity (FC), the maximum amount of water retained in the soil after drainage, and permanent wilting point (PWP), where plants can no longer extract water. The difference between field capacity and the wilting point is available water (AW), and maximum allowable depletion (MAD) refers to the portion of available water that can be depleted without causing stress to the plants, which varies depending on the crop. For further details, please refer to the article “Basics of Irrigation Scheduling”.
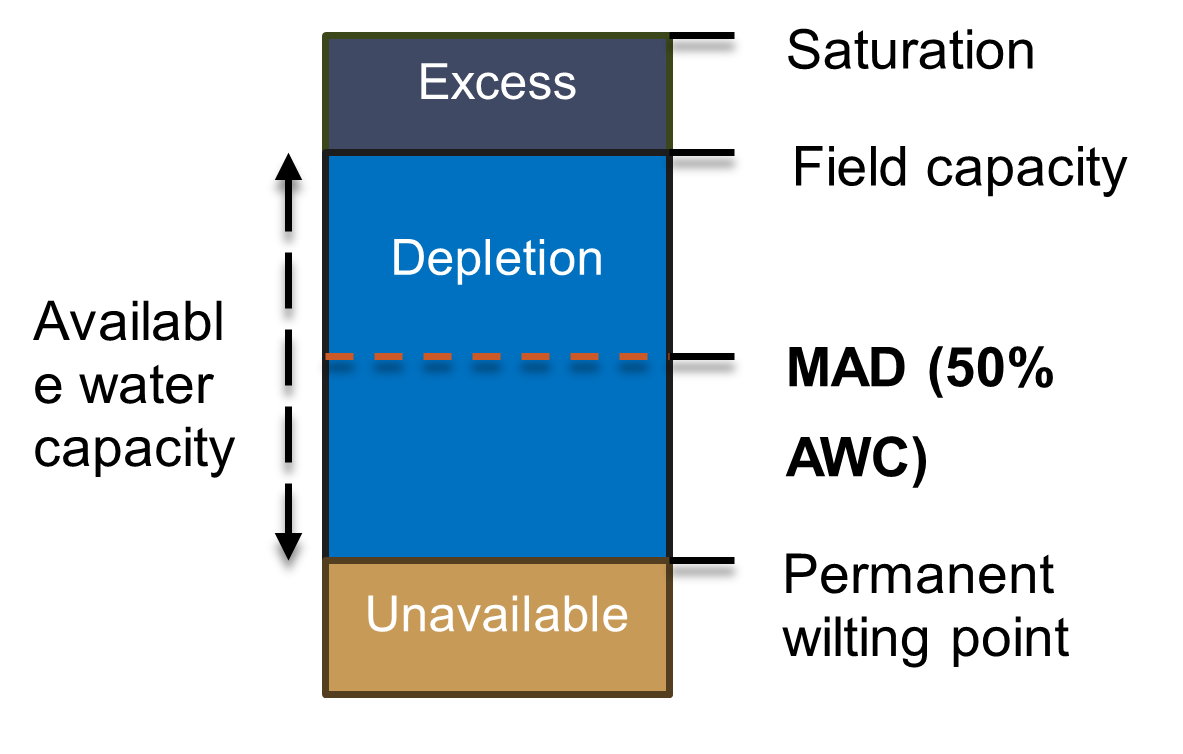
Types of Soil Moisture Sensors
As you evaluate different types of soil moisture sensors, it is essential to determine which option best aligns with your specific needs and objectives. Table 1 summarizes the various sensor types and associated costs. This table is adapted from the works of Sample et al. (2016) and Peters et al. (2013).
Table 1. Soil moisture sensor comparison and their operation mode.
Sensor type |
Mode of operation |
Cost |
---|---|---|
Time Domain Reflectometer (TDR) |
Two parallel rods, known as waveguides, are inserted into the soil. An electromagnetic pulse is transmitted along these rods, and the instrument monitors the reflected signal, measuring the pulse's travel time, which is influenced by soil moisture as it affects the soil's dielectric properties. |
Moderate to High Ranges from $300 to $2,200 |
Frequency Domain Reflector (FDR)/ Capacitance |
It operates by using the soil as a dielectric, measuring the soil’s capacitance, which allows it to store a portion of an electric charge. This is done by running an electrical current through two or more electrodes inserted into the soil. As the soil moisture content increases, its ability to transmit electrical current also increases, which causes changes in the frequency of the wave passing through the soil. |
Low to Moderate Ranges from $50 to $2,000 |
Tensiometer |
It directly measures soil matric potential using a water-filled tube with a porous ceramic cup at one end and a reservoir with a vacuum gauge at the other. As soil moisture decreases, the soil exerts more tension on the water in the tube, causing changes in pressure, which are recorded by the vacuum gauge. |
Low Ranges from $80 to $200 |
Granular matrix sensor |
It indirectly measures soil matric potential by detecting electrical resistance between two electrodes embedded in a granular matrix, which is encased in a gypsum solution and protected by a membrane and external metal casing. A small electric charge is applied to the electrodes, and as soil moisture decreases, the resistance increases. |
Low Starting at $40 per sensor |
Soil moisture sensor reading
For effective irrigation scheduling with TDR and FDR sensors, consider that soil texture, as volumetric water content can vary by site. Table 2 provides field capacity (FC), permanent wilting point (PWP), available water (AW), and recommended trigger points, highlighting that finer-textured soils hold more water than coarser ones. Generally, irrigation is recommended when AW reaches 30-60% depletion, though this threshold may shift based on crop type, growth stage, drought tolerance, and irrigation system capacity. Also, it accounts for forecasted precipitation, evapotranspiration, and irrigation efficiency.
For soil matric potential sensors, such as tensiometers and granular matrix sensors, the University of Nebraska-Lincoln’s Watermark sensor Nebguide EC783 offers a table correlating soil water depletion with matric potential readings, providing water-holding capacities and recommended irrigation triggers for different soil textures. Additionally, the Watermark sensor reading calculator available on SoyWater can be a valuable tool for managing irrigation in corn and soybeans. For more details, refer to Michigan State University’s E3445 Bulletin, improving water use efficiency: Using soil moisture sensors for specific recommendations on using various sensors and installation.
Table 2. Soil water content properties and recommended irrigation trigger points for different soil textures.
Soil texture |
Field Capacity (%) |
Permanent Wilting Point (%) |
Available Water (%) |
Trigger Point Range (%) |
---|---|---|---|---|
Sand |
9.4 |
5 |
4.4 |
6.8 – 8.1 |
Loamy Sand |
12 |
5.7 |
6.3 |
6.2 – 10.1 |
Sandy Loam |
17.9 |
8.1 |
9.8 |
12.0 – 15.0 |
Loam |
31 |
14 |
17 |
20.8 – 25.9 |
Crop water use, or the amount of water required by the crop, can be effectively monitored using soil moisture sensors. For optimal water management, soil moisture sensors should be placed at various depths. Figure 1 illustrates a calculation for determining the irrigation needs of corn, utilizing volumetric water content sensors such as TDR or FDR/capacitance sensors. In this example, a root depth of 3 feet is considered, with soil textures varying across the depth intervals of 0-18, 18-30, and 30-36 inches, specifically loamy sand, loamy sand, and sand, respectively. Soil moisture readings were recorded at depths of 12, 24, and 36 inches. According to the data, the available water for the crop within the root zone is 2.94 inches, and the maximum water-holding capacity is 4.16 inches. Consequently, approximately 1.22 inches of water could be applied to the field, assuming no precipitation is forecast.
Sensor placement considerations
- Sensor Placement: For row crops, sensors should be installed between plants, while for fruits and vegetables, they should be positioned at the center of the root mass.
- Avoidance of Wheel Tracks: Sensors should not be placed near wheel tracks or the edges of lanes to ensure accurate measurements.
- Non-Representative Areas: It is advisable to avoid locations that do not accurately represent the field’s irrigation patterns, such as under end guns, cornering arms, or near the center pivot point.
- Installation Depth: Sensor depth should align with the crop’s root depth. Typical installation depths are at one-third, two-thirds, and the full depth of the crop’s root zone. For example, for corn, sensors would be placed at depths of 12 inches, 24 inches, and 36 inches. Installing additional sensors at varying depths enhances the understanding of soil water movement. The sensor positioned at the bottom of the root zone can assist farmers in determining the optimal timing for irrigation termination based on moisture spikes.
- Multiple Sensor Sets: Utilizing multiple sensors sets within a field can yield valuable data, particularly in areas with variable soil textures, topography, and different crop types.
Soil moisture sensors are a valuable tool for real-time irrigation scheduling, providing essential data to optimize water use. However, selecting the right sensor and ensuring proper placement are crucial for accurate and effective measurements. It’s also important to understand that different sensors may measure soil water content in various ways, so knowing how to properly interpret the data is key to making informed irrigation decisions.
References
Dong, Y., Miller, S. A., & Kelley, L. (2020). E3445 Improving Irrigation Water Use Efficiency: Using Soil Moisture Sensors. Michigan State University Extension.
Sample, D. J., Owen, J. S., Fields, J. S., & Barlow, S. (2016). Understanding Soil Moisture Sensors: A Fact Sheet for Irrigation Professionals in Virginia. Virgina Cooperation Extension.
Peters, R. T., Desta, K. G., & Nelson, L. (2013). Practical use of soil moisture sensors and their data for irrigation scheduling. Washington State University Extension.